Fluid therapy is the most commonly used initial therapeutic intervention in treatment of shock states (e.g. in hypovolemic and distributive types of shock). The approach to fluid resuscitation evolved over the last few decades. For instance, we no longer start with a full “shock dose” of fluids (60-90 ml/kg), and instead, an incremental 10-20 ml/kg boluses are preferred with frequent reassessment of the end-goal perfusion parameters. The standard therapeutic targets include improving heart rate, pulse quality, capillary refill time (CRT), non-invasive or invasive blood pressure and mucous membrane color.
But, how reliable is this approach in assessment of fluid responsiveness? Today, I will explore available evidence and diagnostic tools that can be utilized in evaluation of fluid responsiveness in veterinary and human patients.
Let’s start our discussion with a clinical example.
Imagine that you are presented with a 7 year-old spayed female Labrador who has a history of acute lethargy, anorexia and one episode of vomiting. During your primary survey you notice that she is dull, tachycardic at 200 beats per minute, has poor quality femoral pulses, temperature of 102F, and a Doppler blood pressure of 75 mmHg. Venous blood gas results reveal a high anion gap metabolic acidosis (HAGMA) with a high lactate at 6.9 mmol/L (Reference interval, 0-2.5 mmol/L). You perform an abdominal and thoracic focused ultrasound that is negative for free fluid. By just eyeballing left ventricular (LV) contractility, it appears subjectively normal to increased (hyperdymanic LV), which makes a systolic cardiac failure unlikely. The heart itself appears under-filled and “empty” (i.e. small end-systolic and end-diastolic dimensions) supporting the hyperdynamic appearance of the heart.
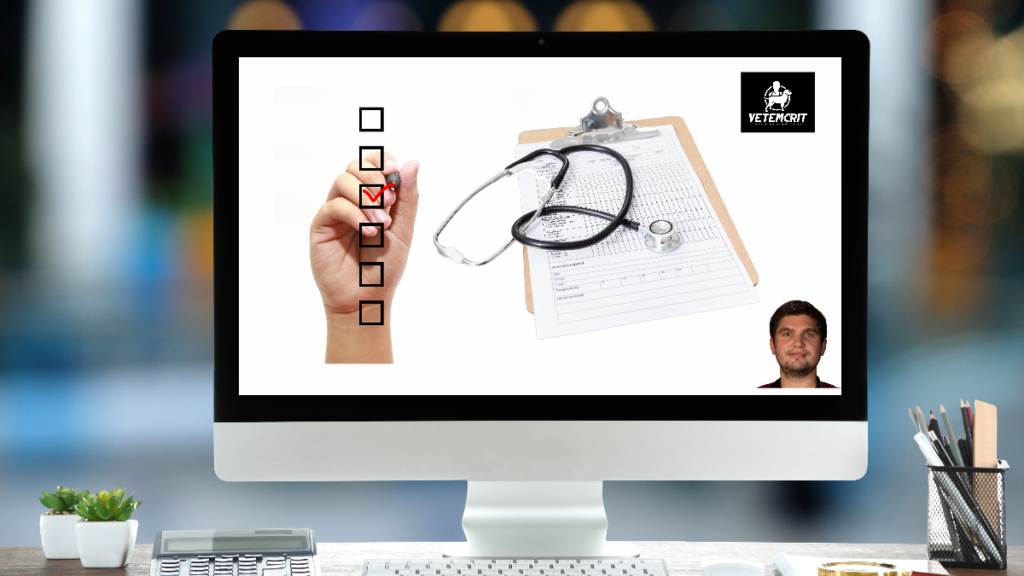
As a result, you feel comfortable giving this dog up to three 20 ml/kg boluses of balanced crystalloid solution with reassessment of your therapeutic targets after giving each bolus. Sixty ml per kg of fluids later, the dog remains dull, the heart rate decreases from 200 to 170 bpm, pulse quality improves somewhat, and systolic blood pressure increases from 75 to 85 mmHg. The serum lactate goes down from 6.9 to 4.9 mmol/L. Overall, the dog remains hemodynamically unstable but all of her perfusion parameters slightly improved.
Is this dog responsive to fluids?
Should we continue bolusing her fluids or should we change our strategy and start vasopressors?
At first glance, it appears that the dog is at least partially responding to fluids, her heart rate is going down, her pulses and BP are getting better, etc. But, can we be certain whether this particular dog is a fluid-responder given the provided information?
By definition, fluid responsiveness is the response of stroke volume or cardiac output to fluid loading or change in preload. In other words, it is a strictly dynamic variable. We have to create a change in preload first, and then, detect a change in stroke volume in order to say if a particular patient is fluid responsive or not.
Based on the growing body of literature in human medicine, it appears that static parameters of cardiovascular performance such as heart rate, blood pressure, CRT, central venous pressure (CVP), end diastolic volume and others are quite inaccurate in assessment of fluid responsiveness.
What is the physiology behind fluid responsiveness?
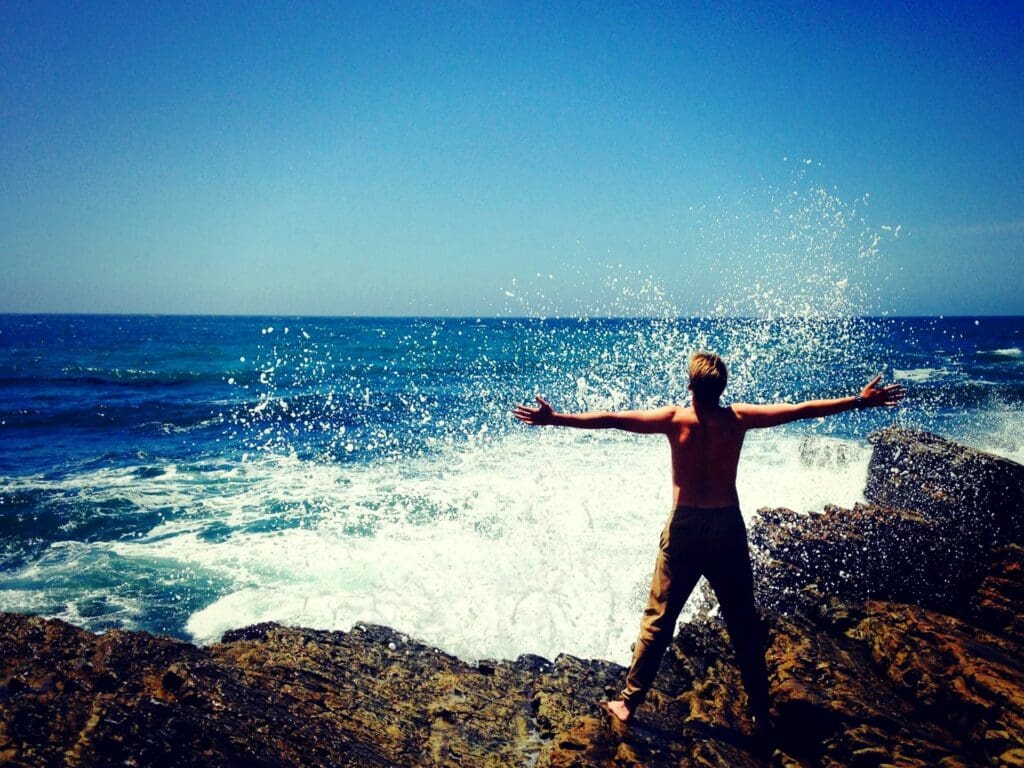
First of all, to become a fluid responder, their right ventricle (RV) must be able to accept the intravenous volume we are giving to them. For a fluid bolus to get from a cephalic vein to the systemic arterial circulation, it should pass through right atrium, tricuspid valve, right ventricle, pulmonary circulation and come back to the left cardiac chambers. If the right ventricle is already overloaded or dysfunctional, the fluid bolus may cause more harm than benefit.
On the other hand, the left ventricle must be able to use this intravenous volume. Even if the right ventricle can accept the fluid bolus, but left ventricular systolic or diastolic function is impaired, there will be no increase in stroke volume. In other words, a fluid-responsive patient should have the RV that is capable of “taking” this fluid and the LV that will use it effectively. Knowing and understanding this concept will help us in assessment of fluid responsiveness.
According to the human critical care review paper that evaluated multiple studies on the topic of fluid responsiveness (Michard et al; Chest 2002), only about 50% of critically ill patients who were perceived as hypovolemic based on static parameters were actually fluid responsive.
What about mean or systolic arterial pressure?
Can changes in arterial blood pressure predict fluid responsiveness? Some studies published in the Journal of Intensive Care Medicine (Pierrakos et al, ICM 2012; Lakhal et al, Ann Fr Anesth Reanim. 2012) found that changes in MAP did not predict an increase in cardiac output from fluid load in people with septic shock. According to this paper, non-invasive systolic arterial pressure (SAP) measurements were only 17% reliable in predicting fluid responsiveness.
Similar results were found in pediatric medicine. Based on a systemic review (Gan et al, Anesth Analg 2013), most static variables such as heart rate and systemic arterial blood pressure did not predict fluid responsiveness in children accurately. In the majority of the studies included in this review, area under the ROC curve (i.e. a fancy statistical test) for heart rate as a predictor of fluid responsiveness was 0.55-0.6, meaning that you can get similar results by flipping a coin.
Why are static variables such as heart rate, pulse quality, mean arterial pressure and CVP so inaccurate in predicting fluid responsiveness based on multiple human studies?
To answer this question, let’s take a look at the well-known Frank-Starling curve (FS curve).
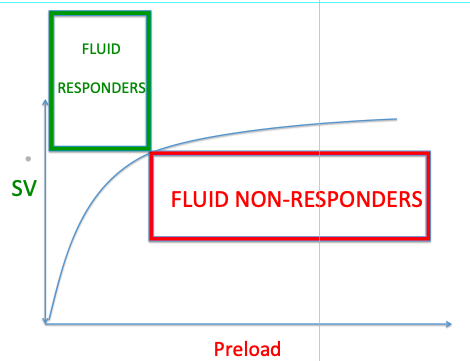
For those who forgot, Frank-Starling curve describes the relationship between the ventricular preload (i.e. how much blood the ventricles are getting filled with by the end of diastole) and stroke volume (how much blood the ventricles pump out during each systole). There is a steep and flat portions of the curve.
If the heart is currently working on the steep portion of the curve, with every bit of increase in preload the stroke volume will significantly increase, which we call “fluid responsive”.
If the heart is working on the flat portion of the curve, increase in preload (e.g. by giving a fluid bolus) will not result in a sensible increase in stroke volume (= fluid non-responder).
A Change in Preload
Taking into consideration this concept, to accurately predict if your patient is a fluid responder or not, we have to generate a change in preload (e.g. give a fluid bolus or change an intra-thoracic pressure), and measure a change in stroke volume. Unfortunately, an inherent limitation of static variables is that they are not able to tell us where our patient is on the Frank-Starling curve. Imagine if somebody asked you to adjust a dose of insulin based on a single blood glucose measurement without generating a blood glucose curve. That is exactly what we are doing when we use static variables to predict whether our patients are fluid responsive or not.
In clinical practice, a change in preload can be generated by giving a fluid bolus or providing positive pressure ventilation. In humans, a passive leg raise test can also be used to deliver an “endogenous” fluid bolus in order to increase a preload. This technique has been validated in anesthetized dogs and pigs as well (Paranjape et al, AJVR 2019; Paranjape et al, Vet Anaesth Analg 2023).
Detection of a Change in Stroke Volume (SV)
On the other hand, a change in stroke volume can be detected by invasive techniques (e.g. thermodilution cardiac output measurement) or by minimally invasive or non-invasive techniques (e.g. pulse pressure variation and echo-derived stroke volume calculation).
A pulse pressure variation (PPV): To apply this method, your patient should be on positive pressure ventilation and have an arterial line placed. As we discussed earlier, to be able to dynamically assess fluid responsiveness, we have to generate a change in preload and measure a stroke volume. In this particular method, the change in preload is created by positive pressure ventilation. During mechanical breath the venous return is predictably diminished due to generation of positive intrapleural pressure that changes a preload.
In patients responsive to increase in preload (those on the steep portion of the FS curve), there is a significant variation in pulse pressure (PP) or stroke volume (SV) during inspiration and expiration. A cut-off of 10-12% in PP or SV variation is commonly used in human medicine.
In one study, the accuracy of PPV and SVV evaluated by area under the ROC curve to predict fluid responsiveness was shown to be 0.84 and 0.94 respectively, which outperformed static variables such as CVP and left ventricular end diastolic area (Cherpanath et al; Neth Heart J 2013). Unfortunately, any spontaneous breaths or even arrhythmias may interfere with these measurements.
Echo-derived stroke volume: This is the most available method. By measuring LV or RV outflow tract diameter and velocity-time integral using a PW Doppler, an echo-derived stroke volume can be calculated before and after a change in preload is generated (by using the continuity equation). This technique can be learned by non-cardiologists and can be performed on spontaneously breathing patients without an arterial line.
Caudal Vena Cava (CVC) Variability
Another ultrasound technique that has been used for evaluation of fluid responsiveness is a variation in caudal vena cava diameter (or IVC in people). This technique will not allow you to directly measure a change in stroke volume, but it can help you to answer the question whether your patient is able to accept more fluids or not. In a spontaneously breathing healthy subject, cyclic variations in pleural pressure, which are transmitted to the right atrium, produce cyclic variations in venous return, which is increased by inspiration, leading to an inspiratory reduction of about 50% in CVC diameter and can be used as an index of volume status.
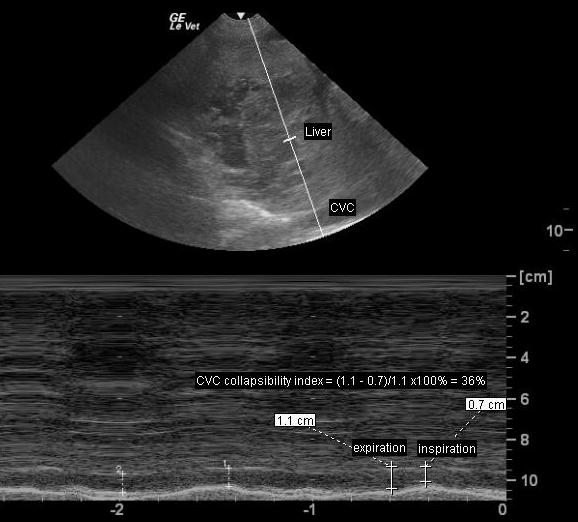
In a patient receiving positive pressure ventilation, the inspiratory phase induces an increase in pleural pressure, which is transmitted to the right atrium, thus reducing venous return. The result is an inversion of the cyclic changes in CVC diameter, leading to increases in the inspiratory phase and decreases in the expiratory phase.
The lack of respiratory variation of CVC during spontaneous or mechanical breathing is suggestive of fluid non-responsiveness or inability to accept a fluid bolus. Hypervolemia and right-sided heart failure are the possible causes of this ultrasonographic finding.
A variation of CVC diameter >50% in spontaneously breathing human patients implies that this patient may be hypovolemic and their RV may accept more fluids. However, this variable cannot predict if the patient’s LV will use this fluid bolus to increase a stroke volume or not.
The Bottom Line
A concept of fluid responsiveness describes an increase in stroke volume in response to an increase in preload. Conventional static variables are incapable of accurately detecting this change. Dynamic indices of fluid responsiveness are preferred, however the majority of them are not validated or routinely used in veterinary species. Hopefully, within the next few years an ongoing research on dynamic evaluation of fluid responsiveness in veterinary species will create a paradigm shift in our fluid resuscitation practices.
References
- Predicting fluid responsiveness in ICU patients: a critical analysis of the evidence. Michard et al; Chest 2002.
- Can changes in arterial pressure be used to detect changes in cardiac index during fluid challenge in patients with septic shock? Pierrakos et al. ICM 2012.
- Brachial cuff measurements of blood pressure during passive leg raising for fluid responsiveness prediction. Lakhal et al; Ann Fr Anesth Reanim. 2012
- Predicting fluid responsiveness in children: a systematic review. Gan et al; Anesth Analg 2013.
- Basic concepts of fluid responsiveness. Cherpanath et al; Neth Heart J 2013.
- Use of a modified passive leg-raising maneuver to predict fluid responsiveness during experimental induction and correction of hypovolemia in healthy isoflurane-anesthetized pigs. Paranjape et al; AJVR 2019.
- Paranjape VV, Henao-Guerrero N, Menciotti G, Saksena S. Volumetric evaluation of fluid responsiveness using a modified passive leg raise maneuver during experimental induction and correction of hypovolemia in anesthetized dogs. Vet Anaesth Analg. 2023 May;50(3):211-219. doi: 10.1016/j.vaa.2023.02.009. Epub 2023 Feb 20. PMID: 36967326.
Thank you for the auspicious writeup It in fact was a amusement account it Look advanced to far added agreeable from you However how can we communicate
Fantastic read! I was especially impressed by the depth provided on the topic, offering a perspective I hadn’t considered. Your insight adds significant value to the conversation. For future articles, it would be fascinating to explore more to dive deeper into this subject. Could you also clarify more about the topic? It caught my interest, and I’d love to understand more about it. Keep up the excellent work!
My brother suggested I might like this website He was totally right This post actually made my day You cannt imagine just how much time I had spent for this information Thanks
Thank you very much for sharing, I learned a lot from your article. Very cool. Thanks.
Hi, I’m Jack. Your website has become my go-to destination for expert advice and knowledge. Keep up the fantastic work!
FinTech ZoomUs Great information shared.. really enjoyed reading this post thank you author for sharing this post .. appreciated
Can you be more specific about the content of your article? After reading it, I still have some doubts. Hope you can help me.